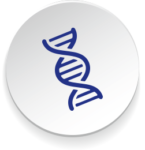
Post Electrophoretic Analysis Articles
PFGE and FIGE
Standard agarose gels can resolve DNA fragments up to 75 kb. Often, analysis of genomic DNA requires resolution of megabase (mb) fragments. Fragments greater in size than one megabase all run at the same rate on agarose gels, (“limiting mobility”), and are therefore not resolved from each other. This reflects the overall mechanism of sieving in agarose (or acrylamide) gels and the rodlike shape of double stranded DNA.
DNA migrating through a gel must find pores large enough to pass through. The size of pore required depends on both the size of the DNA molecule, and its orientation relative to the pore. Molecules oriented perpendicular to their direction of migration present their full length to a gel pore, and thus require a very open gel structure to pass through. The longer a DNA molecule, the more angled it must be to pass through the pores of the gel. Limiting mobility is reached when a DNA molecule can only pass through the gel parallel to its direction of migration, presenting its end to each successive pore. This snakelike progression is called "reptation" and it requires that the DNA maintain itself in as straight a conformation as possible. This highly ordered state is thermodynamically unfavorable, and the DNA will “relax” into a less structured conformation rapidly when conditions permit. Once relaxed, a DNA molecule requires a finite time to reorient itself for further reptation. The longer a DNA molecule, the longer its reorientation will require. In PFGE & FIGE this difference in reorientation time serves as the basis of the electrophoretic separation of megabase sized DNA molecules.
PFGE gels are run in a constantly changing electric field. Originally, gels were subjected to alternating voltage fields oriented at 90° to each other. Current protocols generally employ fields at 120° angles, and more complex systems use 3 or more angled voltages. In all cases, the effect is to force the DNA to continuously re-configure itself to migrate in a new direction. Larger molecules take longer to reorient and therefore make less overall progress through the gel.
FIGE is a special case of PFGE, in which the fields are oriented at a 180° offset, directly opposing each other. In this case, the voltage pulses must be of different strength or duration, so that the DNA makes some net progress through the gel. In FIGE, the timing of the voltage pulses is critical, and must be matched to the reorientation times of the DNA of interest. If the reversing pulse is too short, larger DNA molecules will not reorient, while smaller molecules will reorient and begin to migrate backward. Upon resumption of the forward field, the larger DNA's will be able to resume reptation, while the smaller pieces will rapidly reorient, but then have to make up the distance lost through reverse mobility. The result is that longer DNA's will migrate faster than short pieces. Most often, a progressively longer series of pulses is used, to ensure good resolution over a wide range of sizes. FIGE is easier to use than PFGE, since it can be carried out in a standard horizontal gel apparatus. Its range of size resolution is more limited: up to 2mb as compared to 5mb for PFGE.
A number of devices and systems for PFGE are commercially available. The gel running conditions must be optimized for the sample, the gel apparatus and the size range to be resolved. Parameters include overall pulse lengths, the ratio between forward and lateral pulse length, pulse voltages and the ramp rate between voltages. It is impractical to provide a general protocol which begins to address these variables. The user is referred to the instruction manuals provided with the PFGE units for suggested conditions for their own particular unit.
One of the main challenges in PFGE experiments is to isolate intact DNA in the megabase size range. DNA of this length is easily sheared by turbulence in the solution. Shearing cleaves the DNA at random points, making it impossible to generate discrete bands during restriction digestion. A procedure has been developed in which the cells are lysed and the DNA released within an agarose block, which effectively protects the DNA from shearing forces.
Preparation of High Molecular Weight DNA for PFGE & FIGE Electrophoresis
ENCASE THE CELLS IN AGAROSE BLOCKS
- Prepare a mold to cast the blocks in:
- Tape one end of a plexiglass mold, containing slots of the same size as the wells in the gel.
- Alternatives:
- Cast samples as dots of agarose on a glass or plastic surface, and cut to size.
- Prepare a length of 2mm internal diameter Tygon tubing (-2cm/sample)
- Prepare a solution of 1% agarose in lysis buffer with the following:
- 10mM Tris HCl pH 8
- 100mM EDTA
- 20mM NaCl
- Heat to melt the agarose and cool to 50°C.
- Suspend the cells to be lysed in Lysis buffer at 108 cells/ml, and warm to 50°C.
- Mix an equal volume of agarose and cell suspension, and pipette into molds (or draw up into tubing). Allow to set at 4°C.
- Recover the set plugs into 50ml centrifuge tubes.
LYSE THE CELLS
- Incubate the agarose blocks in 50 volumes of lysis buffer containing 1% Sarkosyl detergent and 0.01% proteinase K, 16-24 hours at 50°C. Remove the supernatant, and replace it with a fresh buffer/Sarkosyl/proteinase K mixture. Incubate for an additional 16-24 hours at 50°C.
- Remove lysis buffer, and wash 3 times at 50°C in 50 volumes of TE + 40 µg/ml PMSF, 1 hour each. (phenylmethylsulfonyl/chloride, a potent proteinase inhibitor). Note: PMSF IS VOLATILE AND TOXIC. USE ONLY IN A FUME HOOD WITH ADEQUATE PRECAUTIONS. PMSF is inactivated after incubation at pH 9 or above for 1 hour.
DIGEST THE DNA
- Equilibrate the blocks in 10 volumes of 1X restriction buffer (optimal buffer for the desired enzyme).
- Remove the buffer and replace it with 3 volumes of 1X restriction buffer containing 50 units of the appropriate enzyme. Incubate for 16 hours at the digestion temperature.
- Wash blocks for 1 hour in 50 volumes of TE @ 4°C.
The blocks may now be loaded driectly into the PFGE wells.
NEXT TOPIC: RNA Electrophoresis
- Using PAGE to Determine Nucleic Acid Molecular Weight
- SSCP Analysis
- Sanger Sequencing
- Sample Preparation for Native PAGE of DNA
- Sample Prep for Denaturing PAGE of DNA
- S1 Mapping
- Run Conditions in Denaturing PAGE
- RNA Mapping
- RNA Electrophoresis
- Ribonuclease Protection
- Restriction Digest Mapping
- Primer Extension
- Preparing Denaturing DNA & RNA Gels
- Preparation of Denaturing Agarose Gels
- Preparation of Agarose Gels
- Pouring Sequencing Gels
- PFGE and FIGE
- PCR Analysis: Yield and Kinetics
- PCR Analysis: An Examination
- Native PAGE of DNA
- Mobility Shift Assay
- Methylation & Uracil Interference Assays
- Maxam & Gilbert Sequencing
- Manual Sequencing
- In Gel Enzyme Reactions
- Heteroduplex Analysis
- Gel Preparation for Native PAGE of DNA
- Gel Electrophoresis of PCR Products
- DNase I Footprinting
- DNA/RNA Purification from PAGE Gels
- DNA/RNA Purification from Agarose Gels – Electroelution
- Differential Display
- Denaturing Polyacrylamide Gel Electrophoresis of DNA & RNA
- Conformational Analysis
- Automated Sequencers
- Analysis of DNA/Protein Interactions
- Agarose Gel Electrophoresis of DNA and RNA – Uses and Variations
- Agarose Gel Electrophoresis of DNA and RNA – An Introduction