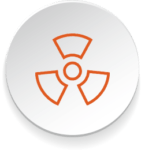
Fundamentals of Liquid Scintillation Counting
Mechanism of Liquid Scintillation Counting
By eliminating the combustion steps needed for gas phase analysis, the introduction of liquid scintillation counting (LSC) reduced the time required to analyze radioactive samples from hours to minutes. For low energy ("soft") β emitters, LSC offers unmatched convenience and sensitivity. LSC detects radioactivity via the same type of light emission events which are used in solid scintillation. The key difference is that in LSC the scintillation takes place in a solution of scintillator, rather than in a solid crystal. This allows close contact between the isotope atoms and the scintillator, which is not possible with solid scintillation. With LSC the short path length of soft β emissions is not an obstacle to detection.
Liquid scintillation cocktails absorb the energy emitted by radioisotopes and re-emit it as flashes of light. To accomplish these two actions, absorption and re-emission, cocktails contain two basic components, the solvent and the phosphor(s). The solvent carries out the bulk of the energy absorption. Dissolved in the solvent, molecules of phosphor convert the absorbed energy into light. Many cocktails contain additional materials to extend their range of use to different sample compositions, but the solvent and the phosphor provide the scintillation of the mixture.
The Role of the Solvent
The solvent portion of an LSC cocktail comprises from 60 - 99 percent of the total solution. When a radioisotope dissolved in the cocktail undergoes an emission event, it is highly probable that the particle or ray will encounter only solvent molecules before its energy is spent. For this reason, the solvent must act as an efficient collector of energy, and it must conduct that energy to the phosphor molecules instead of dissipating the energy by some other mechanism. The solvent must not quench the scintillation of the phosphor, and, finally, the solvent must dissolve the phosphor to produce a stable, countable solution.
Aromatic organics have proven to be the best solvents for LSC. The prototypical LSC solvent is toluene (The solvents used in National Diagnostics scintillation fluids are safer and less toxic than toluene). The π cloud of the toluene ring (or any aromatic ring) provides a target for β-interaction, which captures the energy of the incident particle. This captured energy is generally lost through transfer to another solvent molecule, as toluene has little tendency to emit light or undergo other alternate decay modes. Thus, a β-particle passing through a toluene solution leaves in its wake a number of energized toluene molecules. The energy from these molecules passes back and forth among the solvent ring systems, allowing efficient capture by dissolved phosphors.
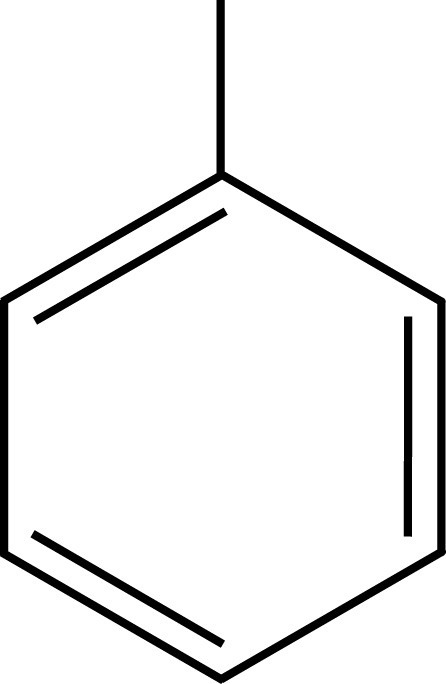
ToluenePXE
Pseudocumene
Solvents employed in liquid scintillation cocktails, such as toluene, pseudocumene, or PXE, possess aromatic rings to absorb the energy of incident radiation

The solvent molecules in a scintillation cocktail absorb a portion of an alpha or beta particle's energy. The energy passes between solvent molecules until the energy reaches a phosphor, which absorbs the energy and re-emits it as light.
The Role of Phosphors (Scintillators)
Phosphors are broadly divided into two classes: primary and secondary scintillators. Included at 0.3-1% of the solution volume, primary scintillators provide the conversion of captured energy to the emission of light. The molecules of scintillator appear to induce a dipole moment in their solvation shell, allowing direct transfer of energy between the scintillator and excited solvent molecules separated by up to 10 other solvent molecules. Primary scintillators must be capable of being excited to a light emitting state by excited solvent molecules, and they must be soluble in the solvent at a sufficient concentration to give efficient energy capture.
Secondary scintillators, or wavelength shifters, were originally included in scintillation cocktails to compensate for the narrow spectral response of early photomultiplier tubes. Most primary scintillators emit light below 408nm, but the response of early photomultiplier tubes drops significantly in this range. A secondary scintillator captures the fluorescence energy of the excited primary scintillator, and re-emits it as a longer wave length signal. The process by which this energy exchange takes place is not clear. (Although the emission spectrum of the primary scintillator and the absorption spectrum of the secondary scintillator generally overlap, the kinetics of the exchange suggest direct contact rather than an emission-absorption event.) While modern phototubes are generally capable of counting the light pulses from the primary scintillator, secondary scintillators have been found to improve efficiency in many cases and are still included in most cocktails.
It has been found that linked benzene rings, rather than larger aromatic systems, generally make superior scintillators. PPO is the most commonly used primary, and Bis-MSB the most common secondary scintillator. Napthalene is somewhat unique, in that it can serve as a low efficiency scintillator and as a solvent, in concert with other organics.
Scintillator | Structure | Emission Wavelength |
---|---|---|
Primary Scintillators |
||
Butyl PBD 2-[4-biphenylyl]-5-[4-tert-butylphenyl]-1,3,4-oxadiazole) Order No. SFC-20 |
![]() |
363nm |
Naphthalene Order No. SFC-40 |
![]() |
322nm |
PPO 2,5-diphenyloxazoleOrder No. SFC-10 |
![]() |
357nm |
p-Terphenyl Order No. SFC-50 |
![]() |
340nm |
Secondary Scintillators |
||
BBQ (7H-benzimidazo[2,1-a]benz[de]isoquinoline-7-one) Order No. SFC-13 |
![]() |
477nm |
Bis-MSB (1,4-bis[2-methylstyryl]benzene) Order No. SFC-90 |
![]() |
420nm |
POPOP (1,4-bis[5-phenyloxazol-2-yl]benzene) Order No. SFC-60 |
![]() |
410nm |
TPB (1,1,4,4-tetraphenyl-1,3-butadiene) Order No. SFC-15 |
![]() |
455nm |
NEXT TOPIC: Liquid Scintillation Signal Interpretation